Subatomic particles are the foundation of everything we observe in the universe, from the tiniest atoms to the vastness of galaxies. The Standard Model of particle physics is the framework that describes these particles and their interactions. But as powerful as the Standard Model is, it leaves unanswered questions about the nature of the universe, leading scientists to propose extensions and new models. Let’s dive deep into the Standard Model, its components, and how the extended model seeks to answer mysteries beyond its scope.
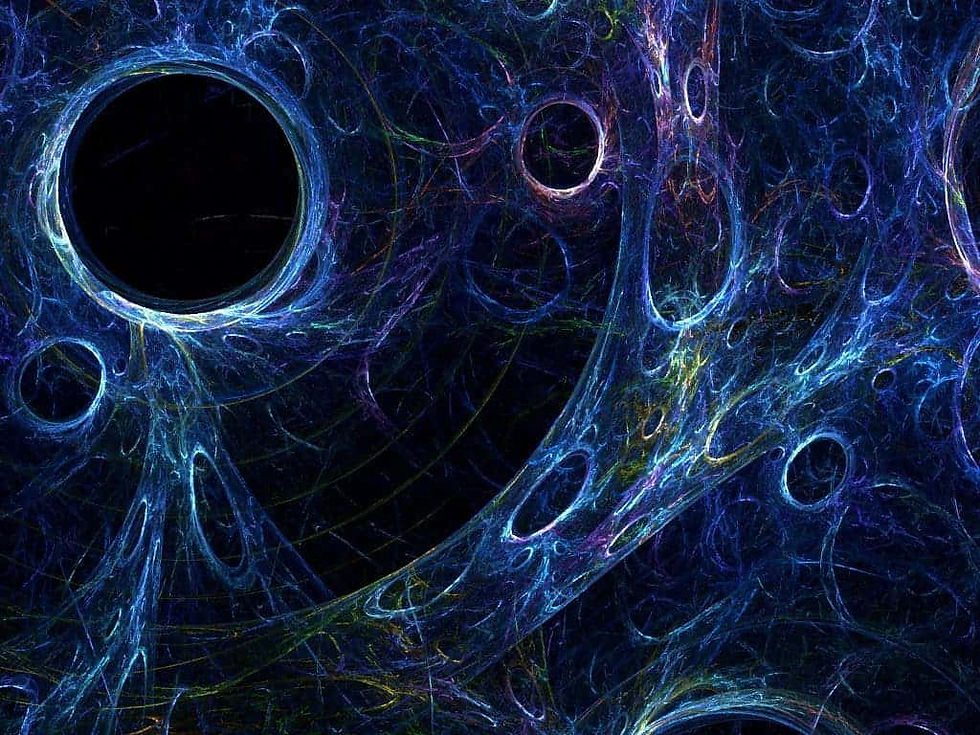
The Standard Model: A Masterpiece of Physics
The Standard Model is a comprehensive theory that categorizes all known elementary particles and describes three of the four fundamental forces of nature: electromagnetic, weak nuclear, and strong nuclear interactions. It is the result of decades of theoretical work and experimental validation.
1. The Particle Zoo: Fermions and Bosons The Standard Model divides particles into two broad categories:
Fermions: The building blocks of matter, which follow the Pauli Exclusion Principle. These are further divided into:
Quarks: Six types (or “flavors”) of quarks—up, down, charm, strange, top, and bottom—combine to form protons, neutrons, and other hadrons.
Leptons: Includes the electron, muon, tau, and their corresponding neutrinos.
Bosons: Force-carrying particles that mediate interactions between fermions. These include:
Photon (γ): Mediates electromagnetic force.
W and Z Bosons: Mediate weak nuclear force.
Gluons (g): Mediate strong nuclear force.
Higgs Boson: Provides particles with mass via the Higgs field.
2. Fundamental Forces in the Standard Model
Electromagnetic Force: Describes interactions between charged particles, mediated by photons.
Weak Nuclear Force: Responsible for radioactive decay and neutrino interactions, mediated by W and Z bosons.
Strong Nuclear Force: Binds quarks together within protons and neutrons, mediated by gluons.
3. Symmetries and Conservation Laws The Standard Model is built upon mathematical symmetries that lead to conservation laws, such as the conservation of energy, charge, and momentum. These symmetries are described by gauge theories, specifically SU(3), SU(2), and U(1).

Successes of the Standard Model
The Standard Model has been extraordinarily successful, with predictions validated by numerous experiments, including:
The discovery of the Higgs boson at CERN in 2012.
Precise measurements of particle interactions and decay rates.
Confirmation of neutrino oscillations, proving neutrinos have mass.
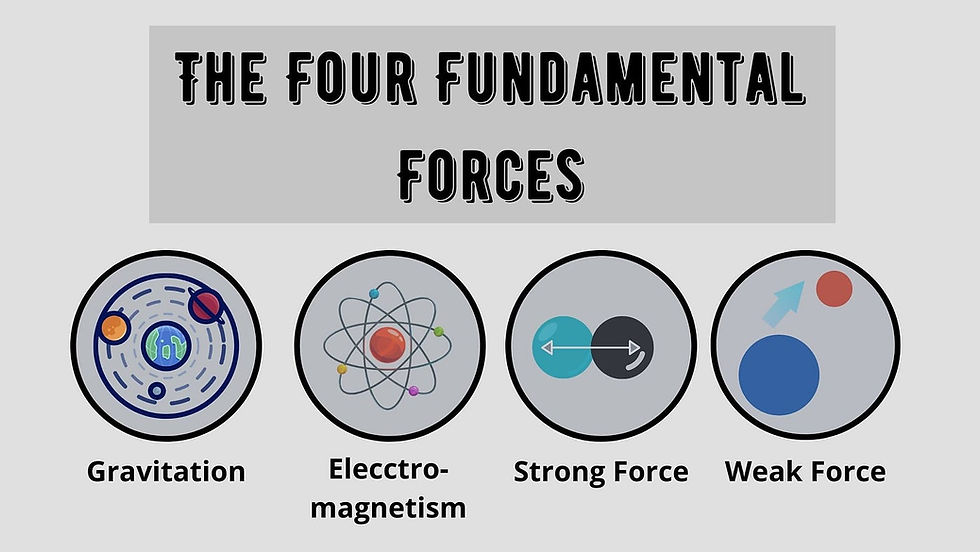
Limitations of the Standard Model
Despite its successes, the Standard Model is incomplete. It does not account for:
Gravity: The fourth fundamental force, described by General Relativity, is not included in the Standard Model.
Dark Matter and Dark Energy: The Standard Model does not explain the mysterious substances and forces that make up 95% of the universe.
Neutrino Masses: The model originally predicted neutrinos to be massless, but experiments show they have a small mass.
Matter-Antimatter Asymmetry: The universe is dominated by matter, but the Standard Model cannot fully explain why.
The Extended Model: Exploring New Frontiers
To address these gaps, physicists have proposed extensions to the Standard Model. These include:
1. Supersymmetry (SUSY) Supersymmetry postulates that every particle in the Standard Model has a superpartner:
Fermions have bosonic superpartners (e.g., selectrons, squarks).
Bosons have fermionic superpartners (e.g., photinos, gluinos). Supersymmetry could explain dark matter, as the lightest supersymmetric particle is a candidate for dark matter.
2. Grand Unified Theories (GUTs) GUTs aim to unify the electromagnetic, weak, and strong forces into a single force at high energies. This involves new particles like X and Y bosons, predicted to mediate proton decay.
3. String Theory String theory proposes that particles are not point-like but rather tiny vibrating strings. It incorporates gravity by predicting the existence of gravitons, hypothetical particles that mediate gravitational interactions.
4. Dark Matter Models Various models propose new particles to explain dark matter, such as WIMPs (Weakly Interacting Massive Particles) or axions.
5. Neutrino Mass Models Extensions to the Standard Model introduce mechanisms like the seesaw model to explain the small masses of neutrinos and their oscillations.
Experimental Efforts to Test Extended Models
Modern experiments are probing the boundaries of the Standard Model and its extensions:
Large Hadron Collider (LHC): Searches for supersymmetric particles, extra dimensions, and evidence of dark matter.
Neutrino Observatories: Facilities like Super-Kamiokande study neutrino oscillations and masses.
Dark Matter Detectors: Experiments like LUX-ZEPLIN aim to directly detect dark matter particles.
Gravitational Wave Detectors: Instruments like LIGO and Virgo may provide indirect evidence for gravitons.
The Future of Particle Physics
The Standard Model represents a monumental achievement in physics, but it is just the beginning. As new experiments and theories push the boundaries of our understanding, the extended model offers tantalizing possibilities for uncovering the true nature of the universe. From dark matter to quantum gravity, the quest continues to answer some of the most profound questions in science.
Works Cited
CERN. "The Standard Model." CERN. Accessed January 5, 2025. https://home.cern/science/physics/standard-model.
Griffiths, David. Introduction to Elementary Particles. Wiley-VCH, 2008.
Particle Data Group. "Review of Particle Physics." Progress of Theoretical and Experimental Physics, 2020.
Hawking, Stephen. A Brief History of Time. Bantam Books, 1988.
"How Particle Accelerators Work." Fermilab. Accessed January 5, 2025. https://www.fnal.gov/pub/science/particle-accelerators.html.
Weinberg, Steven. The Quantum Theory of Fields. Cambridge University Press, 1995.
Comments