Experimenting with Light in 37 Dimensions: A Quantum Interferometry Breakthrough
- professormattw
- Mar 3
- 17 min read
Recent reports in Popular Mechanics and other outlets highlighted a mind-bending experiment where scientists created a photon that “exists” in 37 dimensions . This feat was achieved using a sophisticated interferometer setup to push the limits of the Greenberger–Horne–Zeilinger (GHZ) paradox – a thought experiment that exposes the clash between quantum mechanics and classical “local realism.” Below, we delve into the experiment’s methodology, the evidence for these 37 dimensions, and why the results are exciting for both quantum foundations and theories involving higher dimensions. We’ll also cover how the scientific community has reacted (with both excitement and caution) and discuss future experiments that could build on this achievement.

Interferometer Methodology: Reaching 37 Dimensions
GHZ Paradox in the Lab: The GHZ paradox (named for physicists Greenberger, Horne, and Zeilinger) is a theoretical scenario where quantum mechanics produces outcomes that defy classical logic. In a GHZ setup, entangled particles yield results that cannot be explained by any local realistic theory, leading to contradictions like a calculation where 1 = -1 if one assumes classical behavior . It’s a “no-go” theorem showing that quantum predictions simply can’t fit into our everyday notions of cause-and-effect and independent properties. Until now, GHZ paradoxes had been demonstrated with small systems (typically three entangled quantum bits), but this team wanted to push it to extremes: How non-classical can quantum behavior get? The answer required engineering a system with an unprecedented 37 dimensions – far beyond the minimum of 3 dimensions needed for a basic GHZ paradox .
Time-Domain Optical Interferometer: To achieve a 37-dimensional quantum state, the researchers built a time-domain fiber-optic interferometer – essentially a photonic processor that manipulates pulses of laser light through a complex network of fiber loops, modulators, and beam splitters . Instead of using single-photon sources or matter particles, they used coherent light from a laser (light with a well-defined wavelength and phase). This made it easier to control the system’s properties (like timing and phase) with high precision . Key elements of the methodology included:
• High-Speed Modulation: The laser emitted a train of ultrafast pulses that were modulated in intensity and phase at high speed . By modulating pulses and splitting them, the team created a sequence of light pulses that encoded multiple distinct time slots or modes. In effect, the photon’s wavefunction was spread across 37 time bins, each distinguishable from the others. These 37 time-bin “slices” form the basis of a 37-dimensional Hilbert space (the abstract space of quantum states) .
• Time-Multiplexing and Convolution: The interferometer was designed so that pulses would travel through fiber loops of different lengths, causing some pulses to be delayed relative to others and then recombined. This time multiplexing allowed a single pulse to interact with itself (or with others) at later times, analogous to having many optical paths all folded into the time domain. The paper describes using convolution operations on the time sequences – essentially mixing and overlapping the pulses in carefully controlled ways to create the desired interference pattern among the 37 time slots.
• Beam Splitters and Fiber Network: The setup included multiple beam splitters (some in fiber, some in free space) with tailored transmission/reflection ratios . By splitting and rejoining light in different paths (and using fiber delays), the experiment created complex interference conditions. The detailed experimental diagram (cited by Phys.org) showed blue fiber paths and red free-space paths linking various optical components, with electronic controllers (gray lines) coordinating the timing . Each beam splitter’s ports were tuned so that certain outputs had higher transmissivity (denoted by color coding) to balance the intensities across the many paths . This intricate network acts like a photonic logic circuit, implementing the relationships required by the GHZ paradox scenario.
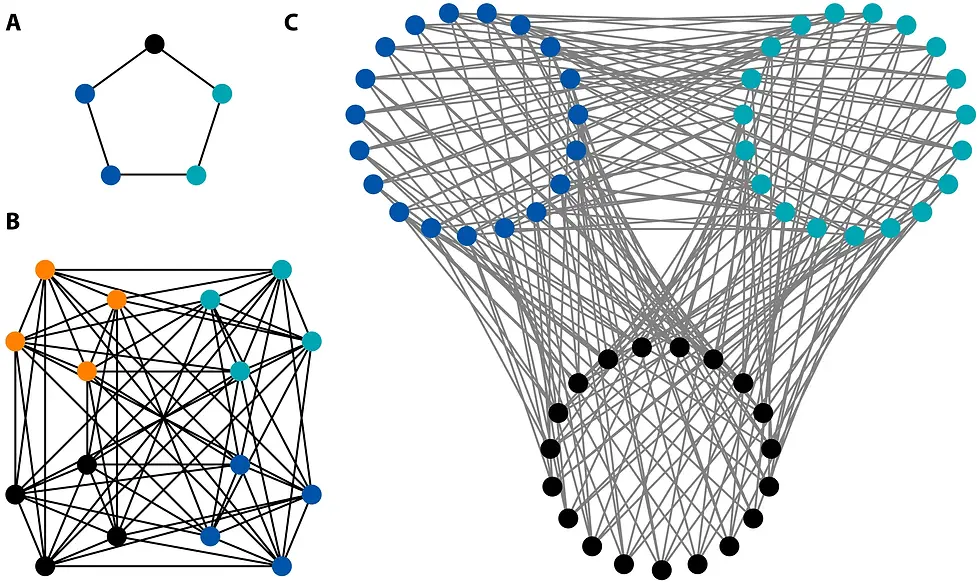
• Entanglement and Contexts: Although the experiment used a laser (which classically is a bright light source), the team effectively created entangled-like correlations between different parts of the light pulse. In the GHZ paradox, you normally have three entangled particles measured in different “contexts” (sets of measurement settings). Here, the “three contexts” were encoded into the optical system’s configuration . Think of each context as a certain choice of how to interfere or measure the light. The researchers worked backward from the desired GHZ correlations to design the optical network that would produce those correlations . In other words, they mathematically determined how 37 distinct states could satisfy a GHZ paradox, and then built the interferometer to realize exactly those states and measurements .
• Homodyne Detection: At the output, instead of simply counting photons, they used homodyne detection – a technique where the outgoing light is combined with a reference laser beam (the “local oscillator”) to measure the amplitude and phase (quadratures) of the light field. This allows extremely sensitive readout of the interference results. By analyzing these signals, the team could retrieve the phase relationships and intensities of all 37 components of the light pulse . Essentially, homodyne detection let them observe the subtle quantum correlations (in the form of interference fringes and amplitude patterns) that correspond to the GHZ paradox predictions.
All of these pieces together formed a “time-domain optical processor” that can choreograph a single pulse of light into 37 interwoven states . It’s as if one photon took 37 different paths simultaneously through the interferometer. This is a massive increase in complexity compared to typical quantum optics experiments, and it was necessary to truly stretch the GHZ paradox to a new regime.
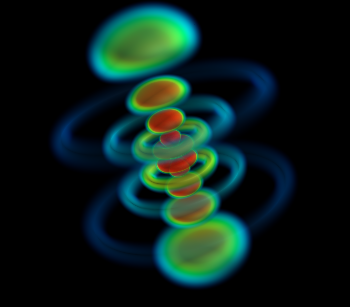
Evidence of Photons in 37 Dimensions
Skeptics might ask: how do we know the photon effectively occupied 37 dimensions? The evidence lies in the correlation patterns and logical contradictions that the researchers observed – patterns that could only be explained if a 37-dimensional quantum state was involved.
• GHZ Paradox Outcomes: The team verified the GHZ paradox in their system by measuring combinations of outputs corresponding to the three different contexts (different interferometer settings). In a classical world of local realism, the outcomes from those contexts can’t all line up consistently if only three dimensions are in play. However, quantum theory predicted that with a 37-dimensional entangled state, the outcomes would show a certain impossible-looking pattern (the GHZ paradox: an “absurd” result that violates any classical assignment of pre-existing values). The experiment indeed “recovered the quantum prediction” for the paradox using their 37-dimensional setup . In other words, the detected interference signals matched the weird quantum correlations to high precision, demonstrating the paradox as expected.
• 37 States as ‘Dimensions’: To put it simply, they found that the light’s behavior could be described as if it had 37 distinct basis states interfering. The ScienceAlert explanation framed it well: the researchers designed a set of relationships between the three contexts that could be solved with 37 states, each representing a different spatial dimension . If fewer dimensions were present, the results wouldn’t add up. The fact that the experiment’s data fit a model requiring 37 independent states is strong evidence that the photon’s quantum state spanned a 37-dimensional Hilbert space . Just as an object in 3D space needs three coordinates, the photon here needed 37 coordinates (amplitudes/phases for 37 basis states) to fully describe its state .
• Beyond Classical Explanation: Crucially, no classical 3D or 4D model could reproduce the observations. The GHZ paradox, especially in this extreme form, has no resolution with “hidden variables” or local realistic assumptions – those would cap out at a much lower complexity. By using 37 dimensions (much more than the minimum required), the team achieved a scenario so complex that it “exceeded all expectations” and emphatically illustrated quantum nonlocality. They essentially showed that even with only three measurement contexts, you can get an outcome that trashes local realism if the system has enough internal quantum states to play with . This is evidenced by the paradoxical results themselves (like the equivalent of the 1 = -1 situation) emerging from the experiment’s measurements, which cannot be mimicked unless those 37-dimensional quantum correlations are real .
In summary, the evidence was a successful demonstration of a logical paradox (the GHZ contradiction) in the lab. Achieving that required and thereby confirmed the presence of a 37-dimensional quantum state of light. As one report put it, “even with just three contexts, our expectations of local realism can be thrown in the bin” by this experiment – a direct sign that an entirely non-classical, high-dimensional quantum description is at play.
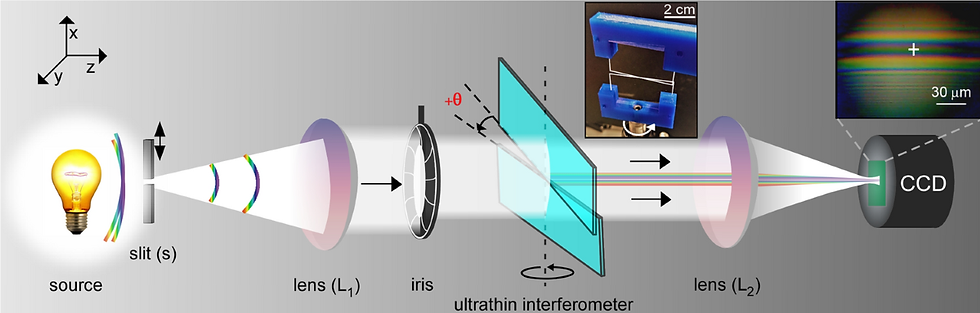
Implications for Higher-Dimensional Physics (and String Theory?)
This experiment is a milestone in quantum foundations, showing that quantum mechanics can exhibit even stronger weirdness than previously known by exploiting high-dimensional states. While the “37 dimensions” here refer to abstract quantum state dimensions (not new spatial directions in our universe), the work resonates with the broader idea of physics in higher dimensions and even offers some indirect food-for-thought for theories like string theory:
• Quantum State vs. Physical Dimensions: It’s important to clarify that the 37 dimensions in this experiment are not extra spatial dimensions popping out of nowhere – they’re dimensions of a Hilbert space, meaning 37 independent quantum states that the photon was in a superposition of . In everyday terms, the photon had 37 “modes” or “reference points” describing it . By comparison, we usually think of objects as having 3 spatial coordinates (x, y, z) and maybe one time coordinate; this photon needed 37 coordinates to describe its full state . The experiment underscores how quantum systems can effectively inhabit many dimensions that aren’t visible in a classical sense. As one explainer noted, it raises the question “do those extra dimensions have any physical meaning?” – are they just mathematical, or could they hint at something real yet hidden? In the context of this photonic experiment, the extra dimensions are a convenient way to describe a complex quantum state, rather than new physical axes in space. However, the successful manipulation of such a high-dimensional state shows that extra degrees of freedom beyond our normal three dimensions can be real and measurable in a quantum system.
• String Theory and Extra Dimensions: Modern string theory posits that the fundamental nature of reality involves 10 or 11 spacetime dimensions (with 6 or 7 extra spatial dimensions beyond the 3 we directly experience) . These extra dimensions in string theory are thought to be compactified (rolled up at tiny scales), which is why we don’t see them in everyday life . The 37-dimensional light experiment doesn’t directly test string theory (it’s not probing hidden spatial dimensions of the universe), but it contributes conceptually to the idea that our physical models may require many dimensions. It provides a concrete example that having more dimensions in a model can yield valid, testable predictions. Just as string theory needs extra dimensions to make the math work for all particle types , the GHZ experiment needed 37 dimensions to make the quantum correlations work. In both cases, although the dimensions are of different types, the lesson is that nature might be described by frameworks far beyond the immediately visible 3D world. This can help the general public and physicists alike become more comfortable with the notion of higher dimensions: if a photon can “live” in 37-dimensional state space and we can observe its effects, perhaps extra spatial dimensions in theories aren’t so outlandish after all.
• Inspiration for Higher-Dimensional Models: Additionally, techniques from this experiment could inspire laboratory simulations of higher-dimensional physics scenarios. For example, some proposals in quantum gravity or higher-dimensional topology could potentially be mapped onto photonic systems with many modes. The experiment shows we have the experimental control to handle many-dimensional entangled states, which might one day allow simulating certain aspects of higher-dimensional quantum models in the lab. While that’s speculative, it’s a door opened by demonstrating this level of control over a photonic system.
In short, the findings reinforce a key theme of modern physics: reality can have more components than meet the eye. We usually deal with three dimensions of space, but quantum mechanics readily expands into high-dimensional configurations. The 37-dimensional light isn’t an alternate universe or a new spatial direction, but it’s a striking reminder that the quantum world’s “dimensionality” far exceeds the classical world’s. It bridges a conceptual gap between everyday intuition and the extravagant requirements of theories like string theory – showing on a small scale that extra dimensions (of a sort) do exist and matter for physics .

Reactions, Reception, and Criticisms
The scientific community has reacted with intrigue to this experiment, seeing it as a major advancement in exploring quantum weirdness. However, there’s also been careful clarification about what the results mean:
• Enthusiasm for Pushing Quantum Limits: Many physicists are excited that this work demonstrated quantum “contextuality” and nonlocality in an extreme regime. Achieving a GHZ paradox with only three contexts but a huge state space shows an unprecedented degree of quantum nonclassicality. As Zhenghao Liu (one of the lead authors) stated, “This experiment shows that quantum physics is more nonclassical than many of us thought” . The team emphasizes that even 100+ years into quantum theory, we may still be scratching the surface of what is possible: “100 years after its discovery, we are still only seeing the tip of the iceberg,” Liu told New Scientist . The published paper itself notes that their results clarify unknowns at a fundamental level and prove quantum mechanics can defy classical assumptions even more strongly than previously demonstrated . Quantum researchers see this as opening new avenues to test the foundations of quantum mechanics and to harness high-dimensional quantum effects for technology.
• Media and Public Fascination: News outlets from Popular Mechanics to ScienceAlert and Phys.org have covered the story, often with sensational-sounding headlines about “light in 37 dimensions” . This generated a lot of public interest, with readers marveling at the idea of 37 dimensions. The positive side is that it draws attention to cutting-edge quantum experiments and stimulates discussion about what “dimensions” mean. Analogies – like the mailbox and Aunt Judy analogy used by ScienceAlert – have been employed to convey the idea of breaking local realism in a humorous way . Overall, the community welcomes the coverage, while working to ensure the core ideas are conveyed accurately.
• Clarifying Misconceptions: Some physicists and science communicators have stepped in to clarify potential misunderstandings. The phrase “37 dimensions” could mislead people into thinking new physical dimensions were discovered in space-time. Articles and discussions (even on social media and forums) have underscored that this is a quantum state phenomenon, not a literal discovery of extra universes or spatial axes. As one analysis put it, the 37 dimensions “refer to the complexity and richness of the quantum state’s description”, rather than extra directions you could point to in a lab . In other words, the photon isn’t zipping around a 37-D room; it’s in a superposition of 37 possible states. This clarification is important to keep the public understanding grounded. It’s a subtle point because in quantum physics, abstract dimensions can have very real effects (as we see here), but they’re not the same as the dimensions in string theory or science fiction. Many experts have taken the opportunity to educate on what Hilbert-space dimensions are, to prevent the spread of the misconception that “scientists found the 5th (or 37th) dimension.”
• Scientific Caution – Complexity and Loopholes: Within the scientific community, the main “criticism” (or rather, caution) is about the complexity and interpretation of the experiment. This was an extremely elaborate setup – 37 dimensions is a record for this kind of quantum-optical demonstration. With complexity comes the challenge of ensuring everything is well-calibrated and that no hidden classical mechanism could mimic the results. The experiment used pulsed laser light (coherent states) rather than single-photon states, which is unconventional for a Bell/GHZ-type test. Coherent light has a classical wave description, so one might wonder: could a clever classical model reproduce these interference patterns? The researchers argue that the answer is no – the specific correlations observed can only arise from genuine quantum contextuality, and the use of coherent light was just a convenient technical tweak to “encode” the quantum problem in an optical form . However, some experts will undoubtedly scrutinize the results to ensure there are no loopholes (analogous to how Bell test experiments are analyzed for detection loopholes or locality loopholes). Given that this is a “proof-of-principle” demonstration, not an outright test of nature’s hidden dimensions, the tone in the community is curious and impressed, but also eager for independent verification or complementary approaches to confirm the findings unequivocally.
• No Immediate Contradiction with Established Physics: It’s worth noting that while the result is counterintuitive (that light can be in 37 states at once), it doesn’t contradict quantum theory – it actually confirms quantum theory’s predictions in a new domain. So there’s no controversy in the sense of disputing the result; rather, it’s seen as a successful, if technically challenging, experiment. Any critique is more about interpretation (as mentioned) or about the next steps: e.g., can this be scaled further, can it be simplified, could there be a more direct way to see the effect, etc. Some might also point out that calling each state a separate “spatial dimension” (as a few articles did) is more metaphor than reality, but that’s a minor semantic quibble as long as one reads the fine print .
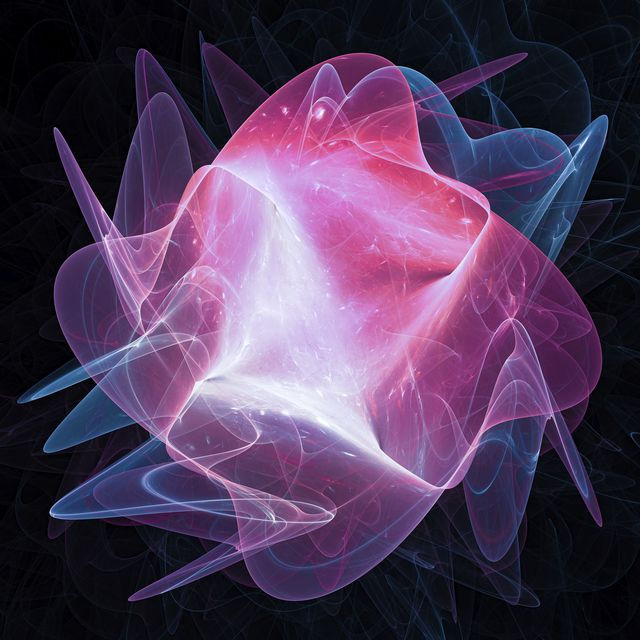
In summary, the reception has been highly positive, with scientists lauding the creativity and precision of the experiment. The main caveat communicated is to understand “37 dimensions” in the proper quantum sense. As long as that’s clear, this result stands as a striking illustration of quantum mechanics’ potential to surprise us. The authors themselves stress humility in the face of quantum physics: we are “only beginning to realize its true potential” . The community, far from dismissing the work, is looking at it as a pioneering step that raises new questions about how much more “quantum strangeness” is out there to be harnessed or discovered.
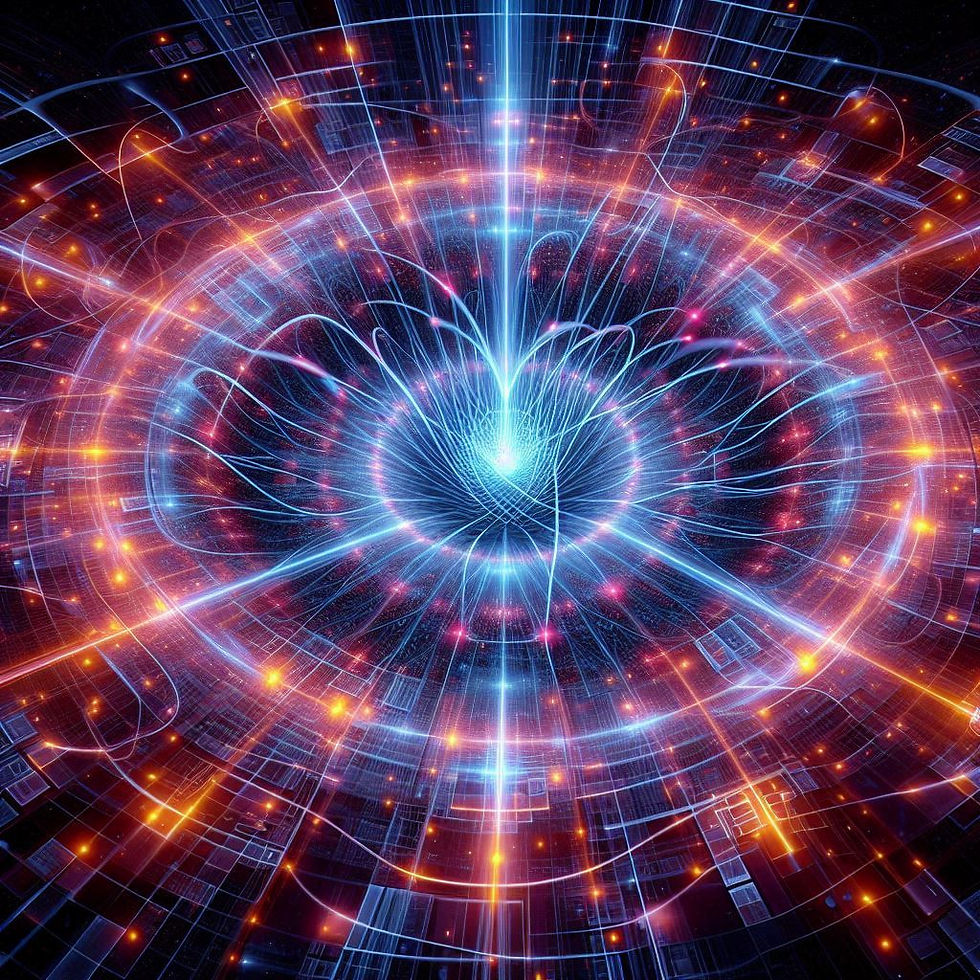
Future Experiments and Applications
This 37-dimensional interferometer experiment opens up many possibilities for future research. Both fundamental physics inquiries and practical quantum technologies stand to benefit from the methods and findings. Here’s a look at what might come next:
• Even Higher-Dimensional Quantum States: The obvious challenge is pushing the dimensionality further. If 37 dimensions revealed new extremes of nonclassical behavior, what about 50 dimensions? 100 dimensions? The current experiment was likely limited by technical factors (loss in fibers, stability of modulators, detection noise). Refinements in optical technology – lower-loss fibers, faster modulators, or perhaps integration on photonic chips – could allow similar GHZ paradox tests with even more complex states. Each jump in dimensionality would test whether quantum mechanics continues to hold (most likely yes, but it’s important to verify) and whether any new paradoxes or phenomena emerge. The authors wrote that their work “opens several avenues for future research” and they “hope [the] findings can be used to build even stronger quantum advantages in high-dimensional systems.” . This hints that they or others might already be thinking about scaling up the dimensionality or finding new protocols that exploit many-dimensional entanglement for tasks like quantum communication or computation.
• Robust Quantum Communication and Qudits: High-dimensional quantum states can carry more information per particle. In quantum communication, for example, using a photon that can exist in 37 states (instead of just 2, like a qubit) means each photon could carry ~$\log_2(37)\approx5.2$ bits of information (i.e. it’s like a “qudit” of dimension 37 rather than a qubit). Such high-dimensional encoding can make communication channels more robust to noise and loss, because even if some dimensions get disturbed, the others still carry information. Future experiments might use similar interferometer setups to create high-dimensional entanglement between two photons (each photon in 37 dimensions, entangled with its partner). This could be useful for quantum cryptography – increasing security and data rates – and for fundamental tests like closing loopholes in Bell’s theorem with high-dimensional entangled states.
• Exploring Strong Contextuality and Quantum Logic: On the foundations side, the team’s paper introduced what they call a “strong form of contextuality in high-dimensional Hilbert space” . Contextuality is the idea that measurement outcomes cannot be pre-assigned independently of other compatible measurements – a very quantum concept. They achieved a GHZ paradox with the minimum number of contexts (three), which is a theoretical milestone in proving strong contextuality . Future experiments could explore other forms of quantum paradoxes, like “rigid” contextuality tests (something the authors mention as a potential direction) . Using similar time-domain optical processors, researchers could implement different mind-bending quantum logic experiments that were previously thought too complex to realize. Each of these tests deepens our understanding of where the boundary between quantum and classical lies – or whether one exists at all.
• Combination of Degrees of Freedom: The experiment focused on time-bin modes of light. But photons have many degrees of freedom: time, frequency, polarization, spatial mode, orbital angular momentum, etc. A natural next step is to combine approaches – for instance, use frequency multiplexing or spatial multiplexing in addition to time multiplexing to reach even higher effective dimensionality. Imagine an interferometer setup that spreads a photon across many time slots and many frequencies simultaneously, creating a truly massive Hilbert space. Such a setup could test whether multiple forms of entanglement can be woven together and still produce coherent GHZ-like paradoxes. It could also simulate more complex quantum networks or even toy models of quantum gravity in the lab by mimicking many interacting components via one photonic system.
• Towards Quantum Computing Applications: A 37-dimensional state could be viewed as a register of quantum information. While one high-dimensional state is not the same as multiple qubits (you can’t necessarily do all the same operations easily), photonic processors like this could be adapted into components of a quantum computer that works with qudits (d-level quantum bits) instead of qubits. Researchers might use similar interferometers to perform high-dimensional quantum gates or algorithms that are more efficient in qudit form. The experiment showed excellent control over a complex quantum state, which is exactly what quantum computing requires. In the long run, the techniques here (fast modulation, multi-path interference, homodyne measurements) might contribute to optical quantum computing architectures.
• Validating Theories with Interferometry: Finally, similar interferometer setups could be used to probe other theoretical ideas. For example, one could test collapse models (theories that modify quantum mechanics to explain why we don’t see superpositions macroscopically) by creating a superposition over 37 paths and seeing if any unexpected decoherence occurs. Or one could attempt to couple such a high-dimensional photonic state to mechanical or atomic systems to test quantum mechanics at larger scales. The flexibility of a time-domain optical processor – which is basically a reconfigurable quantum circuit for light – means researchers could program different experiments on the same device, much like reprogramming a computer. This could accelerate the testing of various quantum hypotheses without needing a completely new setup each time.
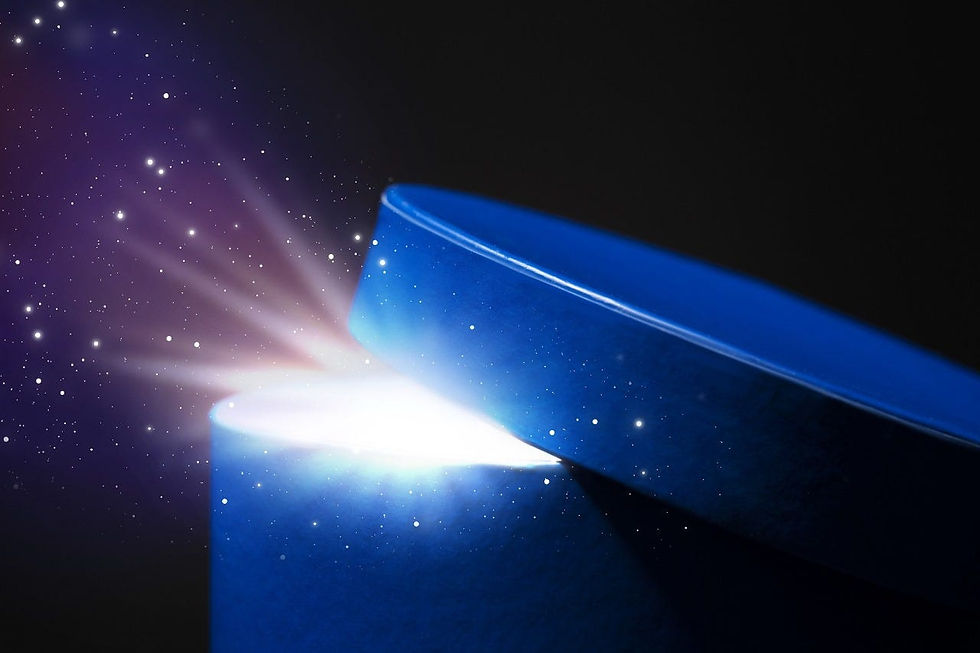
In conclusion, the success of this interferometer experiment is both a proof and a prototype. It proves that quantum mechanics holds strong in a 37-dimensional scenario that utterly lacks a classical explanation . And it’s a prototype for future high-dimensional quantum devices. The researchers themselves expressed hope that this is just the “tip of the iceberg” and that their work will spur “new horizons for future research” . Given the rapid progress in photonic technology, we can expect to see even more creative uses of interferometers to validate and extend these findings. Each new experiment will refine our understanding, either by strengthening the evidence for quantum’s outrageous predictions or by guiding us if any deviation is ever found. So far, quantum theory remains unscathed – and more fascinating than ever – in the face of tests like these.
References:
• Liu, Z.-H., et al. (2025). “Exploring the boundary of quantum correlations with a time-domain optical processor.” Science Advances, 11(5): eabd8080. (See also arXiv preprint: arXiv:2208.07794) – The original journal article detailing the 37-dimensional GHZ paradox experiment, using a fiber-optic time-multiplexed interferometer .
• Yirka, B. (2025). “Extending a paradox: Quantum mechanics experiment measures a pulse of light in 37 dimensions.” Phys.org, Feb 4, 2025. – Summary of the experiment and its significance (quantum vs classical), with an outline of the photonic setup .
• Orf, D. (2024). “Scientists Produced a Particle of Light That Simultaneously Accessed 37 Different Dimensions.” Popular Mechanics, Nov 2023 (online). – Popular science article explaining the result, the GHZ paradox background, and quoting the researchers on the implications of finding “more nonclassical” quantum behavior .
• Coxworth, B. (2025). “Quantum Experiment Reveals Light Existing in Dozens of Dimensions.” ScienceAlert, Feb 2025. – Lay explanation with analogies (mailbox and Aunt Judy) describing how a three-context GHZ paradox was achieved with 37 quantum states, and pondering the meaning of those extra dimensions .
• Hrinko, I. (2025). “Paradoxical discovery: Photons simultaneously exist in 37 spatial dimensions.” Universe Space Tech (Universe Magazine), Feb 10, 2025. – Another accessible write-up emphasizing the difficulty of the experiment and that we usually live in 3+1 dimensions versus the photon’s 37, including quotes from the researchers about the quantum iceberg metaphor .
• Note: Discussions on platforms like Medium and Reddit have also clarified the interpretation of “37 dimensions,” stressing it refers to the photon’s state space, not literal new spatial dimensions . This is an important nuance echoed by scientists to prevent misinterpretation of the experiment’s results.
• For context on higher-dimensional physics theories: String theory requires extra spatial dimensions (typically 10 total dimensions for superstring theory, 11 for M-theory) to unify fundamental forces . These are unrelated to the photonic 37 dimensions, but illustrate how additional dimensions emerge in theoretical physics to “give the strings room to vibrate” and account for all particle types . The successful control of 37-dimensional quantum states in the lab offers a tangential parallel that complex, unseen dimensions can coherently influence observable phenomena – a concept at the heart of higher-dimensional models in physics.
Comments